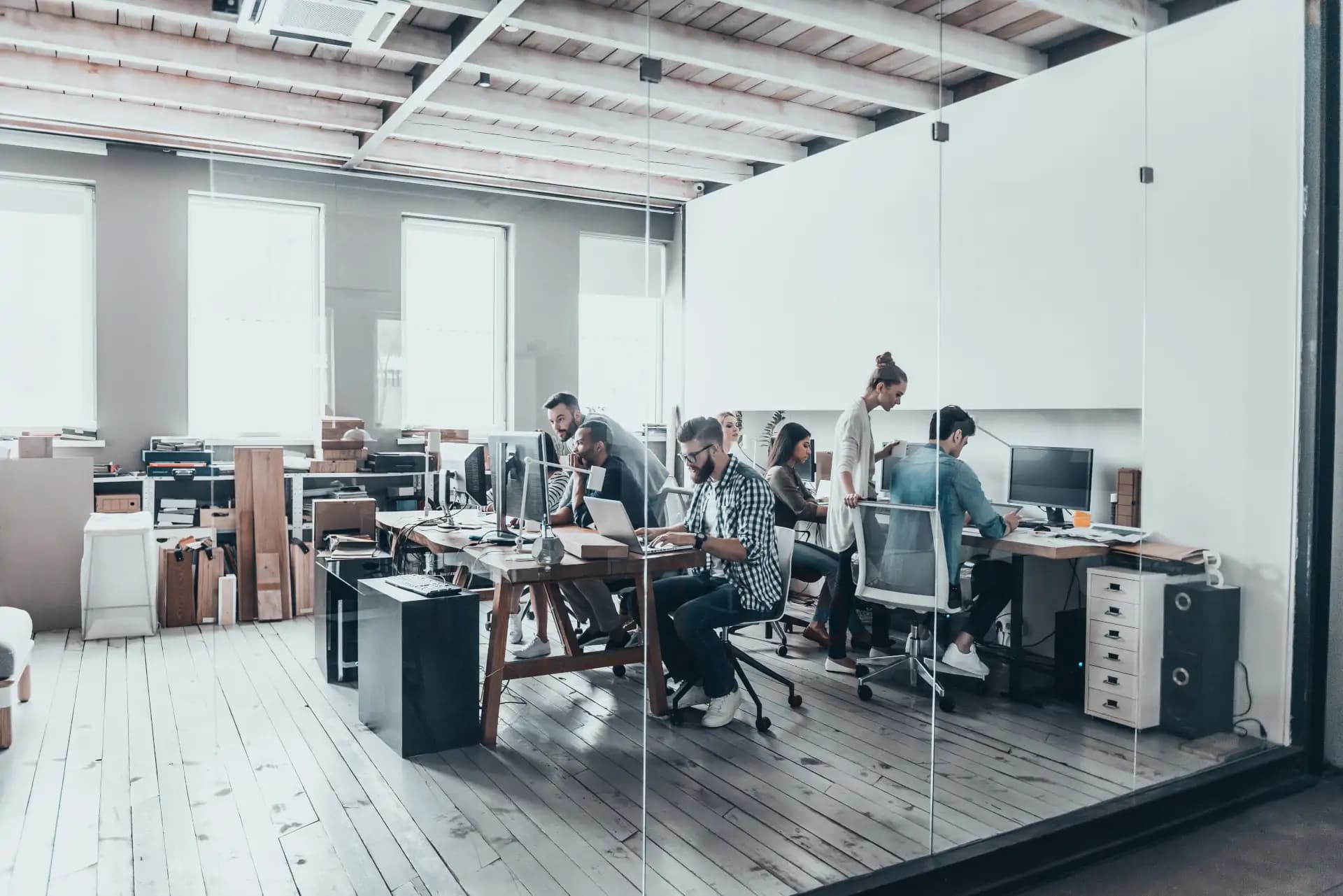
Mastering Thermoplastic Composites with Expertise
The design of components made of fiber-thermoplastic composites is usually closely linked to the processing technology used. For example, due to manufacturing reasons, fiber angles or fiber volume proportions can change during processing and influence the mechanical properties of the entire component. For optimal utilization of these materials, a holistic view of the value chain is a significant advantage. And that’s exactly what we offer our customers:
- We know our manufacturing process. We have developed and optimized the plant technology for manufacturing high-quality products. We therefore know the relevant process parameters and their influence in order to be able to produce high-quality products.
- We know our material. The fibers ensure direction-dependent mechanical behavior in fiber-reinforced plastics. By using thermoplastics as matrix material, this is also combined with highly non-linear material behavior. Knowledge of this mechanical behavior is crucial for precise component design. Together with the Leibniz-Institute for Composite Materials, we have developed a material model that enables us to precisely design the components and maximize the degree of utilization of the fibers used.
- We offer everything from one source. Through the combination of our own manufacturing processes, our own materials and a tailored material model for the design of components, we can offer our customers a unique package for the efficient implementation of components made of fiberthermoplast composites. This special approach not only increases efficiency, but also offers decisive advantages in terms of product performance and market responsiveness.